Abstract: To study the fixation effect of different types of passivators on soil phosphorus, magnesium oxide, ammonium alum and their mixtures in different proportions were added to the soil to study their effects on 0.01 mol·L-1 CaCl2-extractable phosphorus, 0.5 mol·L-1 NaHCO3-extractable phosphorus content and phosphorus components in the soil. The results showed that compared with the unpassivated control treatment, the addition of 0.5% and 2.0% (m/m) magnesium oxide, ammonium alum or their mixture to the soil for 45 days significantly reduced the CaCl2-extractable phosphorus content in the soil. The addition of magnesium oxide or ammonium alum reduced the CaCl2-extractable phosphorus content by 87.8% (0.5%), 98.0% (2.0%), 44.4% (0.5%), and 88.8% (2.0%), respectively, while the passivation effect of the mixture of the two was significantly weaker than that of magnesium oxide. Adding 2.0% magnesium oxide, ammonium alum or their mixture significantly reduced the content of phosphorus extracted by NaHCO3 in the soil, but the effect of the mixture of magnesium oxide and ammonium alum on reducing the content of phosphorus extracted by NaHCO3 in the soil was weaker than that of ammonium alum. Hedley grouping was used to determine the phosphorus components of the soil after 45 days of cultivation. It was found that the addition of magnesium oxide or ammonium alum significantly reduced the content of total phosphorus extracted by H2O and NaHCO3 in the soil, while the addition of ammonium alum significantly increased the content of total phosphorus extracted by NaOH in the soil. The different mechanisms of action of magnesium oxide, ammonium alum and soil active phosphorus are the main reasons why magnesium oxide has a significant effect on fixing CaCl2-P and ammonium alum has a significant effect on fixing Olsen-P.
Vegetables have weak root development and require a high supply of soil phosphorus. Especially in the production of greenhouse vegetable fields, strengthening the application of organic fertilizers and phosphate fertilizers is the key to ensuring the supply of phosphorus [1]. Since the amount of phosphorus absorbed by vegetables is far less than the amount of input, the problem of phosphorus accumulation in vegetable field soil is very prominent. For example, in 2015, the average P2O5 input per crop production in Beijing suburban vegetable fields was 637 kg·hm-2, which is 7 to 32 times the amount of phosphorus absorbed by crops[2]. Considering the crop’s ability to absorb phosphorus, it takes about 9 to 22 years to use 20-50% of the residual soil phosphorus using the depletion method[3]. For vegetable field soil, it takes a long time and cannot quickly reduce the risk of phosphorus leaching. The proportion of organic phosphorus in farmland soil phosphorus is generally less than 20%[4-5], and most of it is inorganic phosphorus in adsorbed, iron-aluminum-bound, and calcium-magnesium-bound states. Therefore, as the amount of soluble phosphate continuously added to the soil increases, after exceeding the mineral adsorption and binding capacity, the effectiveness of phosphorus increases rapidly, even exceeding the soil’s fixed adsorption capacity, resulting in increased mobility in the soil and a rapid increase in the risk of loss to water bodies[6-8].
More than a decade ago, developed countries faced the problem of soil phosphorus accumulation reaching a “saturated” state, and had to take measures such as adding clay minerals, iron-aluminum complexes, and calcium-magnesium complexes to the soil to quickly and effectively fix the active phosphorus in the soil, thereby reducing the movement of soil phosphorus [9-11]. Studies have found that aluminum salts can effectively fix soil active phosphorus [12-13]. Novak et al. [14] added 6.0% aluminum-containing wastewater to different types of high-phosphorus soils, and the soil M3 leached phosphorus decreased by 145~471 mg·kg-1, but the soil pH also decreased significantly. Calcium and magnesium compounds also have strong adsorption, but they are generally alkaline and have a greater impact on soil pH. Michael et al. [15] found that adding 2.0% magnesium oxide to the soil after 4 weeks of incubation can reduce soluble phosphorus by 78.6%, but the soil pH also increased to 9.43. Therefore, different types of mineral passivators have different passivation mechanisms for soil phosphorus. The question raised by this is whether the passivated soil phosphorus is still effective for crops while reducing the risk of environmental pollution? Can different passivators be mixed and applied to fix soil active phosphorus while reducing the impact on soil pH and other nutrient availability?
This experiment took the soil of high-phosphorus vegetable fields in Fangshan District, Beijing as the research object. In an indoor cultivation experiment, 0.5% and 2.0% of ammonium alum, magnesium oxide and their mixture were added to the soil by soil dry weight to explore the effect of magnesium oxide, ammonium alum and their mixture on soil phosphorus fixation within 45 days of cultivation, in order to answer the above scientific questions.
1 Materials and methods
1.1 Experimental materials
(1) Test soil: The calcareous soil for the test was taken from the 0~30 cm soil layer of the vegetable field in Xichang Village, Fangshan District, Beijing. The soil sample was naturally air-dried, and the crop roots, stones and other debris were removed and sieved through a 2 mm sieve for use. Some of its physical and chemical properties are shown in Table 1.

(2) Passivation materials: The magnesium oxide (MgO) (pH value of saturated aqueous solution 10.30) and ammonium alum [NH4Al(SO4)2·12H2O] (pH value of saturated aqueous solution 3.04) used in the experiment were analytically pure reagents from Sinopharm Group. The passivation materials were sieved through a 100-mesh sieve and mixed in the ratio of ammonium alum: magnesium oxide = 1:5, 1:2, 1:1, 2:1, and 5:1 to form a mixture for later use.
1.2 Experimental treatment and method
In field experiments, soil conditioners are generally added at a rate of 2.0% of the soil dry weight [16], but the one-time addition of magnesium oxide and ammonium alum will cause drastic changes in soil pH. The cultivation experiment of this study set up 0.5% and 2.0% (m/m) of the air-dried soil dry weight, 7 kinds of additive material ratios, plus a blank treatment (CK) without the addition of passivation materials, for a total of 15 treatments, with three replicates for each treatment (Table 2). The method of the cultivation test is as follows: weigh 300 g of air-dried soil and the passivation materials required for each treatment (Table 2), mix them on kraft paper, put them into a wide-mouth bottle with a diameter of 8.0 cm and a height of 8.8 cm, add water to adjust the humidity to 60% of the field water holding capacity [the field water holding capacity of the soil is 29.2% (m/m)], and then seal it with a plastic film with small holes and place it in a light-shielded incubator with a temperature of 25°C and a humidity of 70% for cultivation. During the cultivation process, deionized water is added every 3 to 4 days to 60% of the field water holding capacity. Destructive sampling is carried out on the 1st, 15th, and 45th days of cultivation, and the relevant indicators are measured after the soil is naturally air-dried.
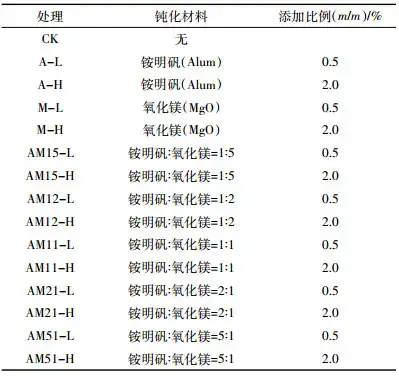
To further explore the transformation of soil phosphorus forms, the fate of reduced water-extractable phosphorus and sodium bicarbonate-extractable phosphorus was studied according to the Hedley phosphorus grouping method. The soil was selected after 45 days of cultivation under the treatment of adding a gradient of 2.0% magnesium oxide, ammonium alum and their mixture. The modified Hedley phosphorus grouping method [17-19] was used to determine the different phosphorus forms in the soil, as follows: 0.5 g of air-dried sample passed through a 2 mm sieve was weighed and placed in a 50 mL centrifuge tube, and extracted with 30 mL of deionized water (H2O-P), 0.5 mol·L-1 NaHCO3 solution (NaHCO3-P), 0.1 mol·L-1 NaOH solution (NaOH-P) and 1 mol·L-1 HCl solution (HCl-P) in sequence. After adding the extract in each step, the mixture was shaken for 16 h (25°C, 200 r·min-1), centrifuged (25 000g, 10 min, 0°C), and the supernatant was collected and filtered through a 0.45 μm filter. The content of inorganic phosphorus Pi was determined by the molybdenum antimony colorimetric method, and the content of total phosphorus Pt was determined by the ammonium persulfate oxidation-molybdenum antimony colorimetric method.
1.3 Determination items and methods
(1) Determination of soil pH: The soil solution was extracted with carbon dioxide-free ultrapure water (soil-water ratio 1:2.5), and the pH was measured using a pH meter.
(2) Determination of soil EC: The soil solution was extracted with carbon dioxide-free ultrapure water (soil-water ratio 1:5), and the EC was measured using an EC meter.
(3) Determination of soil available phosphorus: After extraction with 0.5 mol·L-1 sodium bicarbonate at a soil-liquid ratio of 1:20, the soil was determined using the molybdenum antimony colorimetric method [20].
(4) Determination of water-soluble phosphorus in soil: 0.01 mol·L-1 CaCl2 was extracted at a soil-liquid ratio of 1:5, and then the content of inorganic phosphorus Pi was determined by the molybdenum antimony colorimetric method [21].
(5) The content of inorganic phosphorus Pi was determined by the molybdenum antimony colorimetric method, and the content of total phosphorus Pt was determined by the ammonium persulfate oxidation-molybdenum antimony colorimetric method [17-19].
1.4 Statistical analysis
Data statistics and graphing were performed using Microsoft Excel 2010 software and Sigmaplot 10.0, and variance analysis was performed using IBM SPSS Statistics 20. All data results are expressed as the average of 3 replicates. The Duncan method was used for significant difference analysis, and the significance level was set at α=0.05 (*).
2 Results and analysis
2.1 Changes in soil pH
After adding different types and proportions of passivation materials to the soil, the changes in soil pH are shown in Table 3. After 1 day of incubation, the pH value of the soil treated with ammonium alum decreased, while the pH value of the soil treated with magnesium oxide increased significantly. When only 0.5% of the soil dry weight of magnesium oxide was added, the soil pH value increased from 7.36 to 9.47. When the addition gradient increased to 2.0%, the soil pH value further increased to 9.81. When ammonium alum and magnesium oxide were mixed and added to the soil in different proportions, the soil pH value was between the blank treatment (pH7.36) and the MgO treatment (pH9.47).
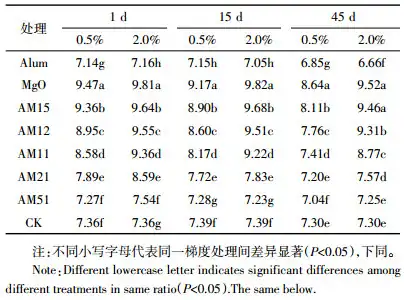
With the extension of the incubation time, the soil pH changed dynamically. The soil pH value of the blank treatment changed little. The soil pH values of the ammonium alum and magnesium oxide treatments decreased with the extension of the incubation time. Compared with the incubation time of 1 day, the soil pH values of the A-L and A-H treatments decreased by 0.29 and 0.5 units respectively after 45 days of incubation; the soil pH values of the M-L and M-H treatments decreased by 0.83 and 0.29 units respectively. The soil pH value decreased the most in the mixture treatments, AM15-L treatment, which decreased by 1.25 units; the least decreased in the AM15-H treatment, which decreased by 0.18 units, which was related to the content of ammonium alum and magnesium oxide in the mixture. The magnesium oxide content in the AM15-H and AM12-H treatments was higher, and the ability to neutralize H+ was stronger, so the effect of decreasing the soil pH value was not obvious. However, the ammonium alum content in other treatments was higher, and the soil pH value decreased more significantly.
2.2 Changes in soil EC value
After adding different types and proportions of passivation materials to the soil, the changes in soil EC value are shown in Table 4. The soil EC value increased significantly after adding ammonium alum and magnesium oxide for 1 day. Adding 0.5% and 2.0% magnesium oxide to the soil dry weight increased the soil EC from 0.78 mS·cm-1 to 1.12 mS·cm-1 and 1.37 mS·cm-1, respectively. Adding 0.5% and 2.0% ammonium alum increased soil EC from 0.78 mS·cm-1 to 2.20 mS·cm-1 and 3.44 mS·cm-1, respectively. In the mixture treatment, soil EC increased with the increase of ammonium alum proportion. Under the 2.0% addition gradient, when ammonium alum: magnesium oxide = 5:1, the proportion of ammonium alum was large, and the EC values of the soil after 1 d, 15 d and 45 d of cultivation were higher than those of the A-H treatment.
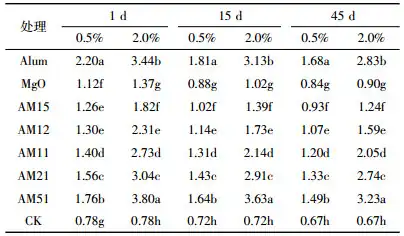
2.3 Changes in soil CaCl2-P content
The changes in soil CaCl2-P content after adding 0.5% of different passivation materials are shown in Figure 1(A). Under the 0.5% addition gradient, all treatments can significantly reduce the soil CaCl2-P content. Compared with the control, the M-L treatment had the most significant effect in reducing soil CaCl2-P. After 1 d, 15 d and 45 d of incubation, soil CaCl2-P decreased by 97.1%, 94.0% and 87.8%, respectively. In the mixture, the AM15-L treatment had the most significant effect in reducing soil CaCl2-P. As the proportion of magnesium oxide in the mixture gradually decreased, the effect of the mixture in reducing soil CaCl2-P weakened, but the effect of the mixture in reducing soil CaCl2-P was still better than adding 0.5% ammonium alum to the soil dry weight.
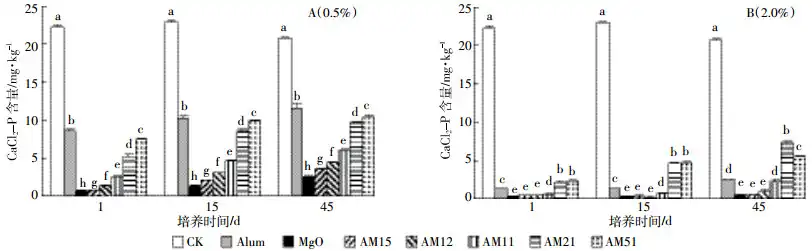
A (0.5%), B (2.0%) represents the addition amount of soil amendments are at 0.5% or 2% of the soil dry weight respectively. the same below
Figure 1 Effect of different amendments on the contents of CaCl2-P in soil at different incubating days
The changes in soil CaCl2-P content after adding 2.0% of different passivation materials are shown in Figure 1(B). Under the 2.0% addition gradient, all treatments can significantly reduce the soil CaCl2-P content, and the effect of reducing soil CaCl2-P is better than the 0.5% addition gradient. Compared with the unpassivated control, the most significant effects of reducing CaCl2-P were in the M-H, AM15-H, and AM12-H treatments. After 1, 15, and 45 days of cultivation, soil CaCl2-P decreased by 99.1%, 98.3%, and 98.2%; 99.0%, 98.8%, and 99.4%; and 98.0%, 97.5%, and 95.7%, respectively. Different from the 0.5% addition gradient, the 2.0% gradient gradually decreased with the proportion of magnesium oxide in the mixture. When ammonium alum: magnesium oxide = 2:1 and 5:1, the effect of the passivator on reducing soil CaCl2-P was not as good as adding 2.0% of soil dry weight of ammonium alum, and even weaker than the M-L, AM15-L, and AM12-L treatments under the 0.5% addition gradient. Moreover, under the 2.0% addition gradient, after 1 d, 15 d and 45 d of cultivation, there was no significant difference in the effect of AM15-H treatment, AM12-H treatment and A-H treatment on reducing soil CaCl2-P. After 45 d of cultivation, there was no significant difference between AM11-H treatment and M-H treatment.
Under the 0.5% addition gradient and the 2.0% addition gradient, the soil CaCl2-P content increased slowly with the extension of the cultivation time. However, the average increase of CaCl2-P under the 2.0% addition gradient was less than that of the 0.5% addition gradient. The treatment with the largest increase under the 0.5% addition gradient and the 2.0% addition gradient was the AM21 treatment, and the soil CaCl2-P content increased by 4.43 mg·kg-1 and 5.18 mg·kg-1, respectively.
2.4 Changes in soil Olsen-P content
The changes in soil Olsen-P content after adding different passivation materials at 0.5% of soil dry weight are shown in Figure 2(A). After 1 day of cultivation, all treatments could significantly reduce soil Olsen-P content, among which the most significant effects were in the A-L, M-L and AM51-L treatments, which reduced Olsen-P by 27.7%, 23.6% and 25.1%, respectively. However, with the extension of cultivation time, the soil Olsen-P content in the M-L, AM15-L, AM12-L and AM11-L treatments increased significantly. After 15 days of cultivation, there was no significant difference between the AM11-L treatment and the blank treatment; after 45 days of cultivation, there was no significant difference between the M-L, AM15-L, AM12-L and AM11-L treatments and the blank treatment. However, the soil Olsen-P content in the A-L and AM51-L treatments did not increase with the extension of cultivation time.
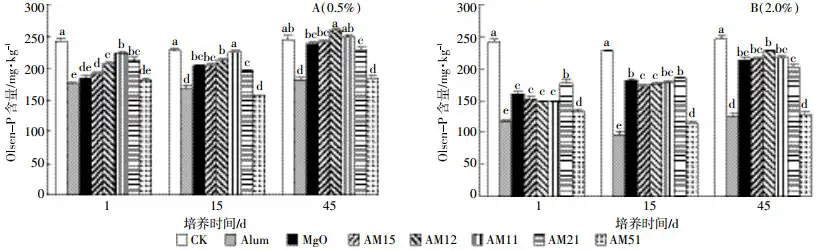
The changes in soil Olsen-P content after adding different passivators at 2.0% of soil dry weight are shown in Figure 2(B). Under the 2.0% addition gradient, all treatments can significantly reduce the soil Olsen-P content, and the effect of reducing soil Olsen-P is better than that of the 0.5% addition gradient. The most significant effects are in the A-H treatment and AM51-H treatment, which reduce the soil Olsen-P content by 51.2% and 45.4%, respectively. Moreover, similar to the 0.5% addition gradient, the soil Olsen-P content in the A-H treatment and AM51-H treatment did not increase with the extension of the incubation time. In the M-H, AM15-H, AM12-H, AM11-H and AM21-H treatments, compared with the incubation time of 1 day, the soil Olsen-P content increased by 32.8%, 40.5%, 53.3%, 45.3% and 15.3% after 45 days of incubation, respectively. However, after 45 days of cultivation, the Olsen-P content in soil of all treatments was significantly lower than that of the control treatment without the addition of passivation materials.
The effect of ammonium alum on reducing soil Olsen-P was stronger than that of MgO, but under the 0.5% addition gradient, after 1, 15 and 45 days of cultivation, as the proportion of ammonium alum in the mixture gradually increased, the passivation agent had a tendency to first weaken and then strengthen after 1, 15 and 45 days of cultivation. This trend also appeared after 45 days of cultivation under the 2.0% addition gradient, which may be related to the change of soil pH.
2.5 Changes in soil phosphorus components
The soil phosphorus forms determined by the modified Hedley phosphorus grouping method are shown in Figure 3. Under the 2.0% addition gradient, after 45 days of cultivation, the H2O-Pt content in the soil treated with the addition of passivation materials was significantly lower than that in the blank treatment without the addition of passivation materials, which is consistent with the results of 0.01 mol·L-1 calcium chloride extraction after 45 days of cultivation. Compared with the control without the addition of passivation materials, after 45 days of cultivation, the A-H, M-H, AM21-H and AM51-H treatments could significantly reduce the content of NaHCO3-Pt in the soil, among which the A-H treatment had the best effect, reducing it by 60.0%, and the effect of reducing soil NaHCO3-Pt showed a trend of gradual strengthening with the increase of the proportion of ammonium alum in the mixture. Compared with the control, the A-H and AM51-H treatments significantly increased the content of NaOH-Pt in the soil, increasing by 289% and 137%, respectively, while the other treatments had no significant effect on the content of NaOH-Pt in the soil. Compared with the control, the effects of each treatment on the content of HCl-Pi in the soil were not significant.
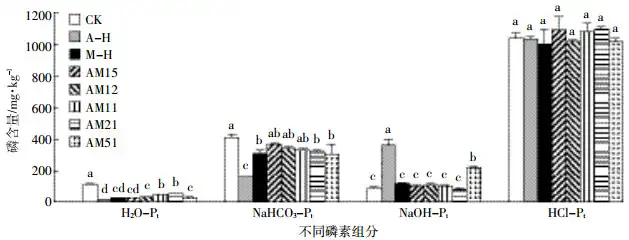
3 Discussion
3.1 Effects of adding magnesium oxide and ammonium alum on soil CaCl2-P
Adding magnesium oxide, ammonium alum or a mixture of the two in different proportions could significantly reduce the content of CaCl2-P in the soil. The results of phosphorus components (Figure 3) showed that after adding ammonium alum, CaCl2-P was converted to phosphorus (NaOH-P) adsorbed on aluminum compounds by chemical adsorption. However, under the condition of equal mass addition, the CaCl2-P value of the treatment with magnesium oxide addition was significantly lower than that of the treatment with ammonium alum addition (Figure 1), indicating that magnesium oxide has a stronger ability to reduce soil CaCl2-P. This result may be related to the larger specific surface area of magnesium oxide under equal mass conditions. The more active groups on the surface of magnesium oxide provide sites for adsorption of phosphorus [22-23], making it difficult for active phosphorus to be leached out by 0.01 mol·L-1 CaCl2 solution.
After 1 day of cultivation, the correlation between magnesium oxide content and the reduction of soil CaCl2-P under the 0.5% addition gradient (0.961**) was more significant than that under the 2.0% addition gradient (0.782*). This indicates that the fixation ability of magnesium oxide on soil CaCl2-P was weakened under the 2.0% addition gradient. At the same time, the fact that the soil CaCl2-P content in the AM51-H treatment (1 g MgO + 5 g Alum) under the 2.0% addition gradient was lower than that in the AM12-L treatment (1 g MgO + 0.5 g Alum) under the 0.5% addition gradient also proved this phenomenon. This is because the amount of ammonium alum in the mixture under the high addition gradient is relatively large, and the H+ generated by the hydrolysis of ammonium alum may dissolve part of the magnesium oxide, thereby reducing the adsorption of magnesium oxide.
At the same time, the results of this experiment showed that except for the AM51-L treatment under the 0.5% addition gradient, as the incubation time increased, the soil CaCl2-P content and soil pH value of other treatments showed a significant negative correlation (Table 5), that is, as the soil pH value decreased, the soil CaCl2-P content increased. This may be because as the pH increased, H2PO4– would be converted to HPO42-, and the solubility of HPO42- is lower than that of H2PO4–, making it more difficult for active phosphorus to be leached out by CaCl2 solution [24]. In the AM51-L treatment, the ammonium alum content was higher, and the soil pH value changed less with time, and the effect on the soil CaCl2-P content was also weak.
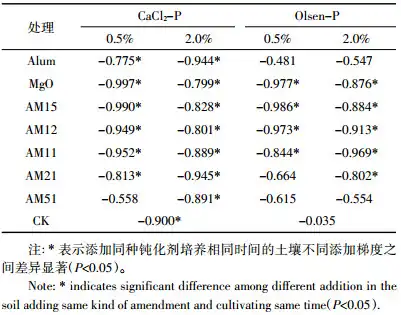
The above results show that the soil CaCl2-P content is related to the properties of magnesium oxide and ammonium alum, as well as the soil pH value. PO43-, HPO42-, and H2PO4– in the soil can undergo coordination reactions with the -OH provided by the surface of magnesium oxide and thus be adsorbed and fixed, but the H+ produced by the hydrolysis of Al3+ will cause OH– to be consumed, thereby destroying the magnesium oxide adsorption site [25].
3.2 Effects of adding magnesium oxide and ammonium alum on soil Olsen-P
Adding magnesium oxide, ammonium alum, or a mixture of the two in different proportions can reduce the soil Olsen-P content. The reason why magnesium oxide reduces the Olsen-P content in soil may be the formation of stable magnesium phosphorus, or it may be that the soil pH increases, and the phosphate ions that are coordinated and adsorbed with the -OH on the surface of magnesium oxide are transformed from unstable single-base coordination to stable double-base coordination [26-27], making it difficult to be leached out by NaHCO3 solution. The reason why ammonium alum reduces the active phosphorus in calcareous soil is mainly due to the adsorption of aluminum hydroxide colloid. The decrease in NaHCO3-P and the increase in NaOH-P content in the phosphorus component results (Figure 3) also indicate the transformation of active phosphorus to stable phosphorus. This is consistent with the results of Moore et al. [28], 6 < pH < 8, Al3+ mainly adsorbs phosphorus by forming aluminum hydroxide colloid.
Unlike the results of CaCl2-P, after 45 days of cultivation, the Olsen-P content of the soil treated with ammonium alum was significantly lower than that of the treatment with magnesium oxide (Figure 2). The reason may be that most of the phosphate adsorbed by magnesium oxide exists on the surface of magnesium oxide, and sodium bicarbonate solution can leach it out.
From 1 day to 45 days of cultivation, the Olsen-P content of the treatment with ammonium alum remained basically unchanged. However, the Olsen-P content of the treatment with magnesium oxide increased from 160 mg·kg-1 to 213 mg·kg-1.
The Pearson coefficient and significance test between soil pH and soil Olsen-P content (Table 5) show that after 1 day of application of the passivator, the Olsen-P content in the soil and the soil pH value also showed a significant negative correlation with the extension of the cultivation time in the treatment with pH>8.0. The decrease in pH value releases the stable magnesium phosphorus on the one hand, and weakens the double-base coordination adsorption of magnesium oxide on phosphorus on the other hand, so that active phosphorus is released.
3.3 Effects of different ratios of magnesium oxide and ammonium alum on soil CaCl2-P and Olsen-P
Magnesium oxide and ammonium alum will bring about changes in soil pH while reducing the content of CaCl2-P and Olsen-P in calcareous soil. Mixing magnesium oxide and ammonium alum in different proportions can effectively alleviate their effects on soil pH (Table 1).
After 45 days of cultivation under the 0.5% addition gradient, the effect of reducing soil CaCl2-P gradually weakened with the decrease in the proportion of magnesium oxide in the mixture. The regression analysis of the mass ratio of ammonium alum to magnesium oxide and the reduction of CaCl2-P showed (Figure 4) that with the increase of the proportion of ammonium alum in the mixture, the reduction of CaCl2-P showed a quadratic nonlinear significant decrease as a whole (P=0.010 4), but there was a trend of gradual increase. After 45 days of cultivation under the 2.0% addition gradient, with the increase of the proportion of ammonium alum in the mixture, the reduction of CaCl2-P first decreased and then increased (P=0.081, Figure 4). Different from the 0.5% addition gradient, under the 2.0% addition gradient, the AM21 treatment reduced the least amount of CaCl2-P, and then gradually increased. Under the condition of equal mass addition, the reduction of CaCl2-P in all mixture treatments was lower than that in MgO treatment, but higher than that in Alum treatment, indicating that magnesium oxide and ammonium alum affect each other after mixing. The results in Figure 1 show that MgO reduces the content of CaCl2-P significantly more than Alum, but under the addition gradient of 0.5%, the addition amount of ammonium alum is small and the effect on soil pH is not obvious. Therefore, as the content of magnesium oxide in the mixture decreases, the reduction of CaCl2-P gradually decreases. Under the addition gradient of 2.0%, the content of ammonium alum in AM21 treatment and AM51 treatment is higher, and the soil pH is significantly lower than that of other mixture treatments. The lower pH destroys the structure of magnesium oxide and reduces the adsorption of magnesium oxide. The results of soil EC value show that the EC value of AM51 treatment is higher than that of Alum treatment, which also indicates that the H+ produced after the dissolution of ammonium alum can dissolve part of magnesium oxide. Therefore, the CaCl2-P content of AM21-H and AM51-H treatments is higher than that of other treatments. After the structure of magnesium oxide is destroyed, the effect of ammonium alum will be prominent, so the ability of AM51-H treatment to reduce CaCl2-P is stronger than that of AM21-H treatment.
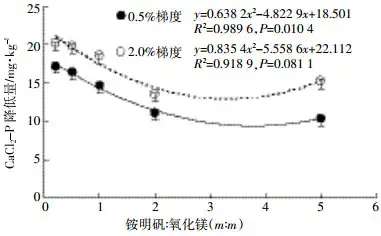
For soil Olsen-P, the results in Figure 2 show that ammonium alum has a significantly stronger effect on reducing soil Olsen-P than magnesium oxide. The regression analysis of the mass ratio of ammonium alum to magnesium oxide and the reduction of Olsen-P showed (Figure 5) that the reduction of available phosphorus increased significantly with the increase of the ratio of ammonium alum to magnesium oxide at the 0.5% and 2.0% addition gradients, but the correlation was significant at the 2.0% gradient (P=0.016), while the correlation was not significant at the 0.5% gradient (P=0.074 2). This is mainly because the amount of magnesium oxide added in the 2.0% addition gradient is higher and the soil pH is higher. The increase in pH may cause OH- and Al(OH)3 to react to form Al(OH)4-[15], which weakens the fixation ability of Olsen-P. Therefore, under the 0.5% addition gradient and the 2.0% addition gradient, the reduction of soil Olsen-P showed a similar pattern, and the AM12 treatment had the worst effect in reducing soil Olsen-P. The soil pH values of the AM12, AM15, and AM11 treatments all exceeded 8.0, and the aluminum hydroxide colloid may undergo alkaline ionization [29-30], and its ability to adsorb soil active phosphorus decreased. There was no significant difference between the AM15-H, AM12-H, and AM11-H treatments.
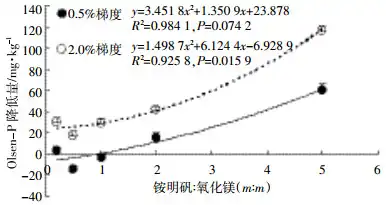
Although the ability of the mixture treatment to reduce soil CaCl2-P and Olsen-P was weaker than that of the single addition of magnesium oxide or ammonium alum, the mixture with a suitable ratio could not only significantly reduce the soil CaCl2-P and Olsen-P content, but also effectively alleviate the drastic changes in soil pH (Table 1).
4 Conclusions
(1) Both magnesium oxide and ammonium alum can significantly reduce the soil CaCl2-P content. After 45 days of cultivation, the 0.5% addition gradient of magnesium oxide and ammonium alum reduced the soil CaCl2-P content by 87.8% and 44.4%, respectively. The 2.0% addition gradient had a stronger effect on fixing Olsen-P than the 0.5% addition gradient. The ability of the two substances to reduce soil CaCl2-P content was weaker than that of magnesium oxide alone.
(2) The ability of ammonium alum to fix soil Olsen-P was stronger than that of magnesium oxide. After 45 days of cultivation, the 0.5% addition gradient of magnesium oxide and ammonium alum reduced the soil Olsen-P content by 3.2% and 13.2%, respectively. The 2.0% addition gradient had a stronger effect on fixing Olsen-P than the 0.5% addition gradient. After 45 days of cultivation, the AM12 treatment had the strongest ability to maintain soil Olsen-P.
(3) Magnesium oxide is a substance that can significantly reduce the water-soluble phosphorus content in soil without affecting the active phosphorus content in soil.