Cobalt is an extremely important strategic metal, mainly used in cemented carbide, ceramic glazes, battery materials, high-temperature alloys and magnetic materials. In recent years, as the country vigorously develops the new energy industry, the cobalt smelting industry has developed vigorously, and the market demand for cobalt raw materials has become increasingly strong.
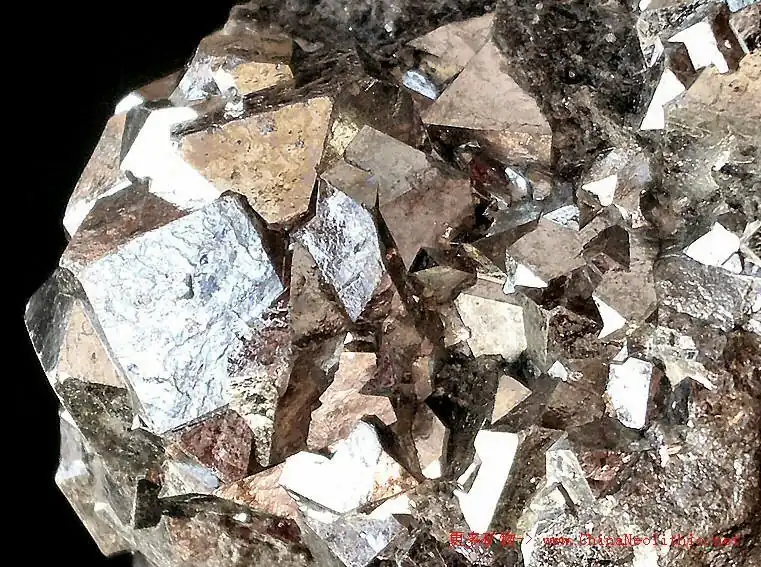
The Democratic Republic of Congo in Africa is rich in copper and cobalt resources, and its cobalt reserves account for nearly 50% of the world’s total reserves. In recent years, more and more cobalt smelting and production companies in my country have built local factories in the Democratic Republic of the Congo to process and produce crude cobalt salts to reduce production costs and raw material transportation costs. It has gradually become an industry trend for crude cobalt salt to replace the import of cobalt concentrate.
The currently commonly used crude cobalt salt production and preparation process has a series of shortcomings such as unsatisfactory impurity removal, high unit consumption of auxiliary materials, and low product cobalt grade. There is an urgent need to develop new processes or optimize the existing crude cobalt salt production process to reduce production cost, improve product quality and enhance product competitiveness.
This article uses a cobalt-containing low-copper raffinate from the Democratic Republic of the Congo as raw material, and prepares high-grade crude cobalt hydroxide products through a process of impurity removal, one-stage cobalt precipitation, two-stage cobalt precipitation, filtration and washing, and flash evaporation and drying. Different from the usual return of the second-stage cobalt underflow to the impurity removal or leaching process to ensure product quality, in this paper, all the second-stage cobalt underflow is returned to the first stage of precipitated cobalt as reaction seed, thus greatly improving the recovery rate of precipitated cobalt and increasing the amount of crude cobalt. The output and comprehensive recovery rate of cobalt hydroxide production products, and its cobalt content reaches more than 39%.
1 Raw materials, equipment and test methods
1.1 Production raw materials
The cobalt-containing raffinate used in production is a low-copper cobalt sulfate solution obtained from a copper-cobalt mine in the Democratic Republic of Congo after grinding, leaching, extraction and other processes. Its main chemical composition is shown in Table 1.

Table 1 Main chemical components of cobalt-containing low-copper raffinate/(g·L-1)
1.2 Main production of auxiliary materials
Quicklime: industrial grade, the proportion of -74μm particle size is not less than 80%, and the main content of active calcium oxide is not less than 75%.
Sodium metabisulfite: industrial grade, the proportion of -74μm particle size is not less than 70%, and the main content is not less than 93%.
Magnesium oxide: industrial grade, the proportion of -74μm particle size is not less than 90%, the main content is not less than 95%, and the activity is not less than 85%.
Compressed air: industrial grade, air pressure not less than 0.4MPa.
1.3 Sample analysis instruments and equipment
X-ray fluorescence spectrometer, flame atomic absorption spectrophotometer, inductively coupled plasma spectrometer, potentiometric titrator, electronic balance, digital pH meter and acid-base titration table, etc.
1.4 Test principle
The treatment process mainly consists of impurity removal, cobalt precipitation and other processes. Impurity removal is accomplished by adding a certain proportion of sodium metabisulfite slurry and compressed air to the low-copper cobalt-containing raffinate to adjust the reaction redox environment and oxidize the Fe2+ that is difficult to hydrolyze and precipitate into Fe3+ that is more easily hydrolyzed and precipitated, and at the same time, quicklime slurry is introduced The material adjusts the pH value of the solution to achieve the purpose of removing most of the iron ions. In addition, impurity ions such as Cu and Mn can also be partially removed during the impurity removal process. Cobalt precipitation is mainly done by adding active magnesium oxide slurry to control the pH value of the reaction, so that the Co ions in the solution are precipitated as Co(OH)2 precipitate, and then through the filter press slurry washing, flash drying and other processes to obtain a water content of about 8 % cobalt hydroxide dry product.
1.4.1 Impurity removal reaction
The relevant chemical reactions involved in the impurity removal reaction are as follows:
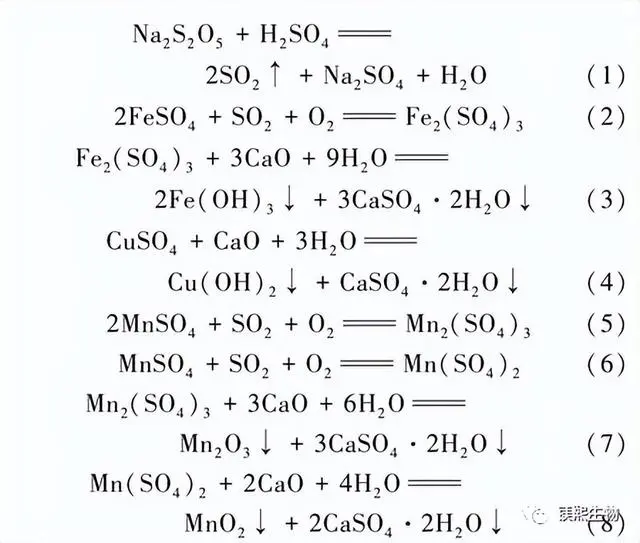
1.4.2 Cobalt precipitation reaction
The relevant chemical reactions involved in precipitating cobalt are as follows:

2 Test results and discussion
2.1 Impurity removal process conditions
2.1.1 Effect of limestone slurry concentration on impurity removal effect
Set the impurity removal time to 5h, pH=4.0, and potential to 420mV. The effects of quicklime concentration on the impurity removal effect and cobalt ion loss rate are shown in Figures 1 and 2 respectively. It can be seen from Figure 1 that the preparation concentration of quicklime has little effect on the removal effect of impurity ions. As the concentration increases, the iron ion removal rate stabilizes at more than 99%, and the manganese ion removal rate basically fluctuates between 35% and 42%. The copper ion removal rate fluctuates between 50% and 60%.
It can be seen from Figure 2 that as the concentration of quicklime preparation increases, the loss rate of impurity-removing cobalt also gradually increases. When the concentration reaches 30%, the cobalt loss rate reaches 12.6%. This is because as the preparation concentration of quicklime increases, the cobalt loss rate per unit time increases. The more quicklime auxiliary materials are added to the space, it is easy to cause the impurity removal reaction to be locally too alkaline, and a large amount of cobalt ions precipitate out instantly. At the same time, the generated cobalt hydroxide precipitate is wrapped in calcium iron slag and cannot be dissolved back into the impurity removal process. solution, resulting in the loss of cobalt ions during the impurity removal process. The main ion loss occurs in the impurity removal process, so a lower concentration of quicklime should be selected. However, the low preparation concentration of quicklime will bring greater challenges to the system water balance and the processing capacity of the auxiliary material conveying equipment. Taking comprehensive considerations into account, it is appropriate to select a quicklime concentration of 15%.
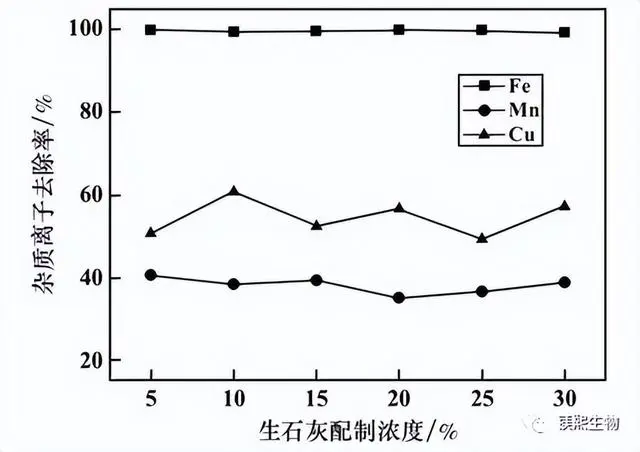
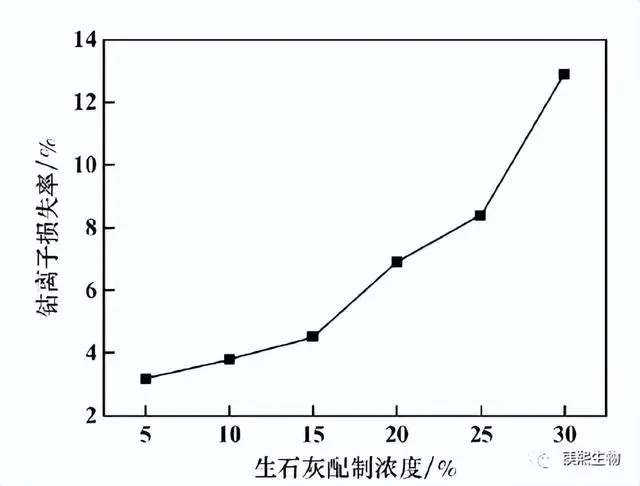
2.1.2 Effect of reaction time on impurity removal effect
The effect of quicklime concentration 15%, pH=4.0, potential 420mV, and reaction time on the impurity removal effect is shown in Figure 3. As can be seen from Figure 3, first, as the reaction time increases, the impurity ion removal rate gradually increases. The iron, copper, and manganese ion removal rates tended to be stable at 5h, 4h, and 6h respectively. Taking comprehensive considerations into account, it is appropriate to select 5 hours for the impurity removal reaction time.
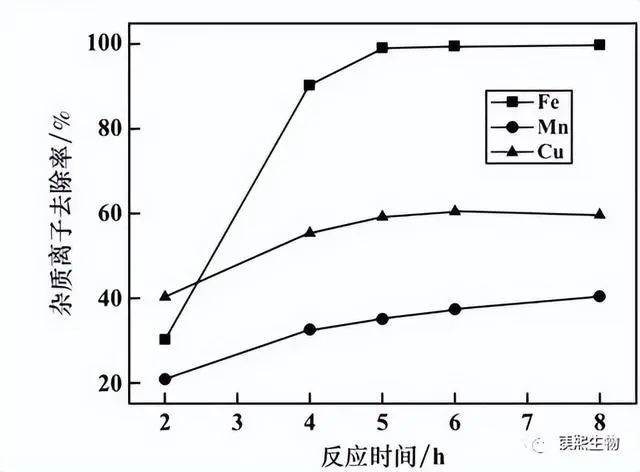
2.1.3 Effect of reaction pH value on impurity removal effect
The effect of quicklime concentration 15%, reaction time 5h, potential 420mV, and pH value on the removal rate of impurity ions is shown in Figure 4. As can be seen from Figure 4, the impurity ion removal rates in the early stages of the reaction increase as the pH value increases. When pH=3.5, the iron removal rate is 92.3%. When pH=4.0, the iron removal rate tends to be stable and remains above 99.1%. Continuing to increase the pH value has little effect on iron removal. When pH=4.5, the copper ion removal rate reaches 60.4%, and when pH=5.5, the removal rate reaches 99.8%. When pH=5.0, the manganese ion removal rate tends to be stable and remains at about 41.5%. An excessively high pH value will cause a greater loss of cobalt ions. In principle, the lower the pH value, the better while ensuring a good enough impurity removal effect. Taking comprehensive considerations into account, it is appropriate to select a pH value of 4.5 for the impurity removal reaction.
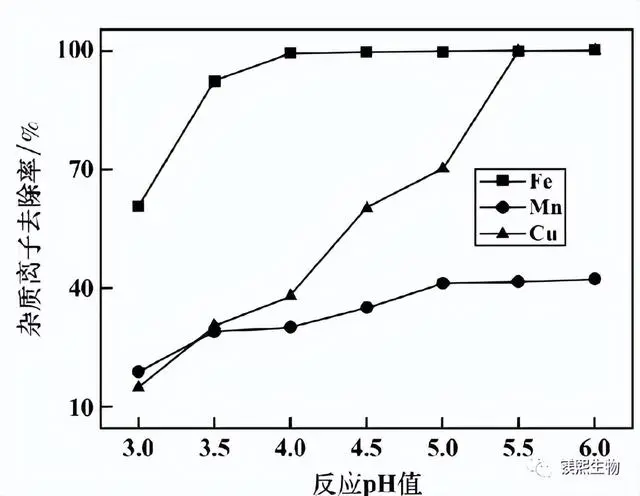
2.1.4 Effect of reaction potential on impurity removal effect
The quicklime concentration is 15%, the reaction time is 5 hours, and the pH is 4.5. The influence of potential on the impurity removal effect is shown in Figure 5. As can be seen from Figure 5, the impurity ion removal rate gradually increases as the reaction potential increases. When the potential rises above 420mV, the iron removal rate tends to be stable, reaching more than 99.2%. When the potential is above 450mV, the manganese ion removal rate does not change significantly and remains at about 41%. All things considered, the impurity removal reaction potential is controlled at around 420mV.
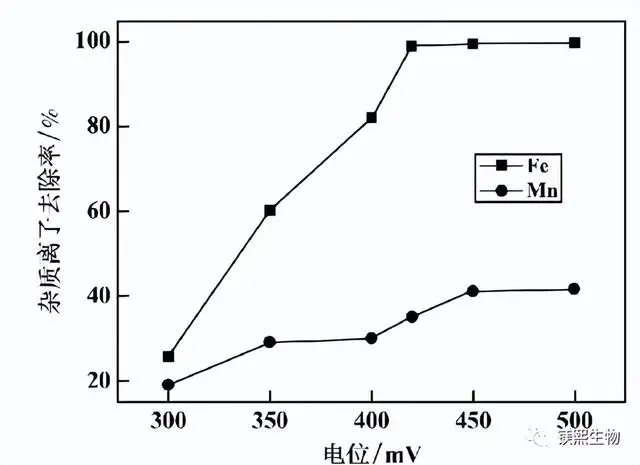
2.2 First stage cobalt precipitation process conditions
2.2.1 Effect of pH value on the quality of first-stage precipitated cobalt hydroxide
The reaction time was 5 h, the magnesium oxide addition amount (tMgO/tCo) was 0.8, and the effect of pH value on the quality of cobalt hydroxide in the first stage of precipitated cobalt was investigated. The results are shown in Figure 6.
It can be seen from Figure 6 that the Co grade of cobalt hydroxide initially increases with the increase of pH value, reaches the maximum value (45.6%) at pH=8, and then gradually decreases. This is because as the pH value gradually increases, the alkalinity in the solution becomes stronger and stronger, more and more cobalt ions precipitate, and the proportion of cobalt ions in the product becomes higher. However, when the pH value rises to a certain level, Afterwards, the cobalt ions in the solution were completely precipitated, and other impurity ions began to precipitate in large quantities. At the same time, the magnesium oxide auxiliary materials added in large quantities were not completely consumed and entered the cobalt hydroxide product, resulting in a decrease in the cobalt grade in the cobalt hydroxide. The changing trend of magnesium impurity ions in the product is just the opposite. At the beginning, as the pH value gradually increased, a large amount of cobalt ions in the solution precipitated. The magnesium ions in the magnesium oxide slurry were basically completely consumed, and a smaller amount was transferred to the product. When the pH value reaches above 8, there is an excess of magnesium oxide in the solution, and a larger proportion of magnesium oxide enters the cobalt hydroxide product, causing the magnesium impurity content in the product to gradually increase. Comprehensive consideration, it is appropriate to select a pH value of about 8.0 for the cobalt precipitation reaction in the first stage.
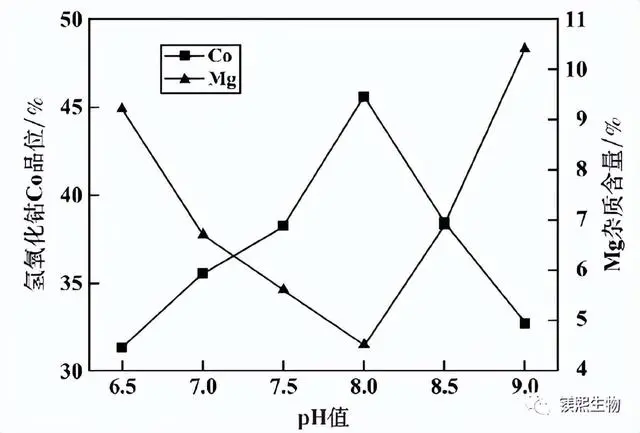
2.2.2 Effect of reaction time on the quality of one-stage precipitated cobalt hydroxide
The reaction pH=8.0, the magnesium oxide addition amount (tMgO/tCo) is 0.8, and the effect of reaction time on the quality of cobalt hydroxide is shown in Figure 7. As can be seen from Figure 7, the Co grade of cobalt hydroxide gradually increases with the extension of the reaction time, and becomes stable after the reaction time reaches 6 hours; the impurity magnesium content gradually decreases with the extension of the reaction time, and becomes stable after the reaction time reaches 8 hours. This is mainly because as the reaction time prolongs, more and more cobalt ions precipitate out of the solution, and at the same time, the wrapped and underutilized magnesium oxide gradually dissociates and re-participates in the cobalt precipitation reaction, thus ultimately gradually reducing the magnesium content in the product. Improved product cobalt grade. Comprehensive consideration, it is appropriate to select a period of cobalt precipitation reaction time of 6 hours.
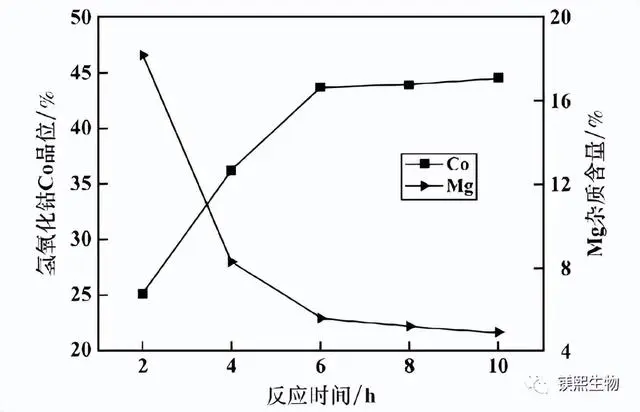
2.2.3 Effect of magnesium oxide addition amount on the quality of first-stage precipitated cobalt hydroxide
The reaction pH=8.0, the reaction time is 6h, and the influence of magnesium oxide addition amount (tMgO/tCo) on the quality of cobalt hydroxide is shown in Figure 8. It can be seen from Figure 8 that the cobalt hydroxide Co grade first gradually increases with the increase in the magnesium oxide addition amount. However, when the magnesium oxide addition amount reaches 1.0, the cobalt hydroxide Co grade reaches the maximum value, and then increases with the magnesium oxide addition amount. increase and decrease. The magnesium impurity content first gradually decreased as the magnesium oxide addition amount increased. When the magnesium oxide addition amount was 1.0, the magnesium impurity content was the lowest, and then began to gradually increase. This is mainly because when the amount of magnesium oxide added is insufficient, as magnesium oxide is gradually added, a large amount of cobalt ions begin to precipitate out of the solution. At the same time, the added magnesium oxide is replaced and consumed, leaving less residue in the precipitate. However, when magnesium oxide is added When the amount reaches above 1.0, as the addition amount continues to increase, magnesium oxide becomes more and more excessive, and the magnesium oxide that cannot be completely consumed directly enters the cobalt hydroxide precipitation, resulting in lower and lower cobalt grades and magnesium impurity content. Getting higher and higher. Comprehensive consideration, tMgO/tCo=1.0 is appropriate for the first-stage cobalt precipitation reaction.
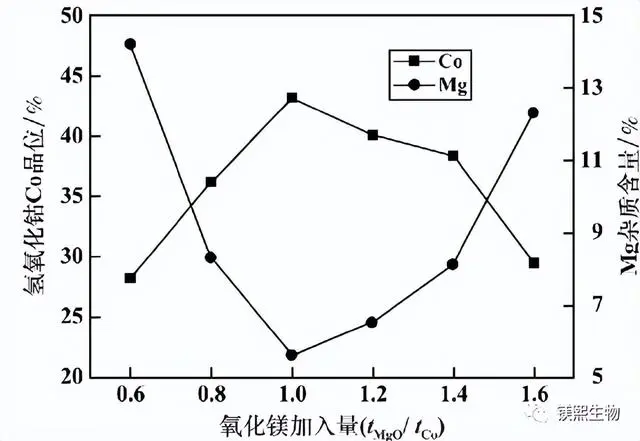
2.3 Second stage cobalt precipitation process conditions
2.3.1 Effect of pH value on the quality of second-stage cobalt slag
The reaction time was 4 hours, and the effect of pH value on the cobalt quality of the second-stage cobalt precipitation slag was examined. The results are shown in Figure 9. As can be seen from Figure 9, when pH=9.0, the Co content of the second-stage cobalt slag reaches 34.2%. Then as the pH value gradually increases, the cobalt content continues to decrease, while the magnesium oxide content increases sharply. This is because the raw material of the second-stage cobalt precipitation is the liquid after the first-stage cobalt precipitation, and the concentration of cobalt ions in the solution is generally low. In the second-stage cobalt precipitation process, the cobalt ions that have not been completely precipitated in the first-stage cobalt precipitation must continue to precipitate, which must be higher. The pH value means that magnesium oxide must be partially excessive. However, if the pH value is too high, impurity ions such as Mn and Mg will precipitate in large quantities, which will lead to a sharp decrease in the Co content of the second-stage cobalt precipitation slag. At the same time, the content of impurities such as magnesium will rapidly decrease. rise. Comprehensive consideration, the pH value of the second-stage cobalt precipitation is selected to be around 9.0.
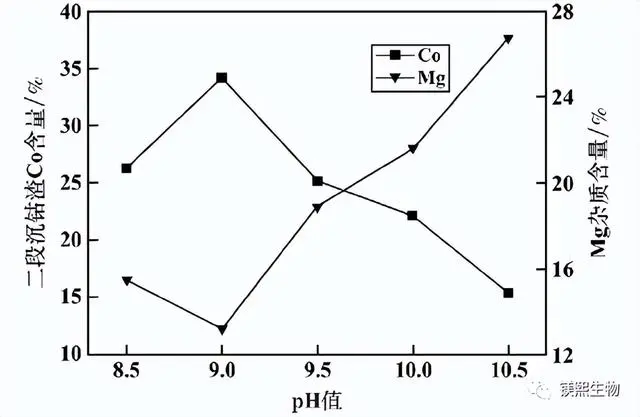
2.3.2 Effect of reaction time on the quality of second-stage cobalt slag
The reaction pH=9.0, and the effect of reaction time on the quality of the second-stage cobalt slag is shown in Figure 10. It can be seen from Figure 10 that as the reaction time increases, the Co content of the second-stage cobalt precipitation slag increases, and at the same time the Mg impurity content decreases. The reaction time reaches more than 3 hours, and the Co content and Mg impurity content of the second-stage cobalt precipitation slag tend to be stable. . Based on comprehensive considerations, the reaction time of the second stage Co precipitation is 3 hours.
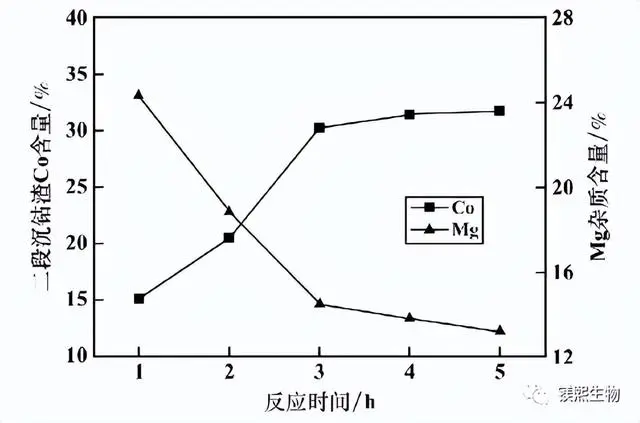
2.4 Precipitated cobalt products prepared under optimized process conditions
Through the above single factor experiment, the suitable impurity removal process conditions are: quick lime concentration 15%, reaction time 5h, reaction pH=4.5, potential 420mV; the suitable process conditions for first-stage cobalt precipitation are: reaction pH=8.0, reaction time 6h, oxidation The magnesium addition amount (tMgO/tCo) is 1.0; the suitable process conditions for the second-stage cobalt precipitation are: reaction time 3h, reaction pH=9.0; and return all the second-stage cobalt precipitation slag to the first-stage cobalt precipitation process as reaction seed crystal. A verification experiment was conducted under this optimized process condition, and the analysis results of the cobalt hydroxide sample obtained are shown in Table 2. It can be seen from Table 2 that the Co grade of cobalt hydroxide reaches 45.60% under the optimized conditions of the first-stage cobalt precipitation; the Co content of the second-stage cobalt precipitation slag reaches 34.20% under the optimized conditions of the second-stage cobalt precipitation; all the cobalt slag of the second stage is returned to the first stage for crystal seeding. The Co content of the final product also reaches 39.65%, fully meeting the product sales requirements.
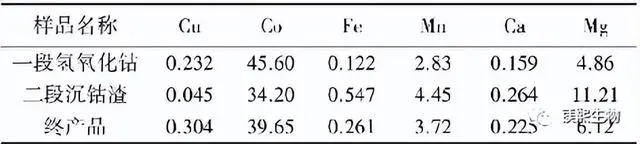
3Conclusion
1) The optimized production process conditions for impurity removal reaction are: quicklime concentration 15%, reaction time 5h, reaction pH=4.5, potential 420mV. The iron removal rate under these optimized conditions is 99.5%.
2) The optimized process conditions for one-stage cobalt precipitation are: reaction pH=8.0, reaction time 6h, and magnesium oxide addition amount (tMgO/tCo) 1.0. The Co grade of cobalt hydroxide prepared under these optimized conditions reaches 45.6%.
3) The optimized process conditions of the second-stage cobalt precipitation are: reaction time 3h, reaction pH=9.0. The Co content of the second-stage cobalt precipitation slag obtained under these optimized conditions reaches 34.2%, and all the second-stage cobalt precipitation slag is returned to the first-stage precipitation. Cobalt is used as the reaction seed crystal, and the Co content of the final cobalt salt product reaches 39.65%.