Abstract: In order to save production costs and reduce the sintering temperature of SiC ceramic membrane support, SiC and ρ·-Al2O3 were used as the main raw materials, basic magnesium carbonate (BMC, added mass fractions of 0, 0.5%, 1% and 1.5%) was used as a sintering aid, and the in-situ reaction-bonded SiC support was prepared by pressureless sintering at 1300-1420℃. The effect of BMC addition on the performance of the support was studied. The results show that the addition of BMC intensifies the surface oxidation of SiC, reduces the in-situ reaction bonding temperature (<1300℃), promotes the formation of necks between SiC particles, and improves the strength of the sample; when the BMC addition amount gradually increases from 0.5% to 1.5%, the apparent porosity of the sample after sintering at different temperatures gradually decreases; the optimal addition amount of BMC sintering agent is 1.0% (w), and the room-temperature flexural strength of the porous SiC support prepared after sintering at 1360℃ is 25.3MPa, which is 2.7 times that of the one without sintering agent. After 5 times of wind-cooled thermal shock at 1050℃, the room-temperature flexural strength retention rate is more than 90%, and the room-temperature flexural strength after thermal shock is more than 19MPa, which can meet the index requirements of membrane support for high-temperature flue gas purification.
Ceramic membrane support is a porous material that plays the role of loading the membrane layer and providing mechanical strength. SiC porous ceramics have good application value as membrane supports in filtration and separation, especially in high-temperature gas-solid separation, due to their high strength, chemical corrosion resistance, low thermal expansion coefficient and excellent thermal stability.
Since the sintering temperature of pure SiC ceramics exceeds 2000℃, some people have developed technologies to reduce the sintering temperature, such as forming a binding phase by chemical reaction at a lower temperature by adding a binder. Among them, adding aluminum-containing micropowder can achieve in-situ reaction to form mullite bonding at a temperature above 1400℃. In addition, doping a small amount of MgO, CaO, SrO, Y2O3, La2O3, V2O5 and CeO2 can reduce the mullite temperature. For example, adding 1% to 5% (w) Y2O3 can reduce the in-situ reaction temperature from 1450℃ to 1350℃; adding V2O5 can reduce the in-situ reaction temperature even more. However, the cost of adding Y2O3 and V2O5 is high. In this work, cheap basic magnesium carbonate (BMC) is used as a sintering aid, and ρ·-Al2O3 is still used as the mullite aluminum source to study the effect of the addition of sintering aids on the sintering behavior and properties of in-situ reaction combined porous SiC ceramics.
Experiment
1.1 Raw materials and sample preparation
The main raw materials used in the experiment are: w(SiC) = 98.89%, black SiC with a medium particle size of 150 and 1.46μm respectively; w(Al2O3) = 86.2%, d50 = 5.2μm ρ-Al2O3; w(MgO) = 48.3%, d50 = 5.8μm basic magnesium carbonate (BMC); w(PVA) = 10% PVA solution.
The ingredients were prepared according to m(coarse SiC)m(fine SiC)m(ρ-Al2O3)=94:1:5, and BMC with mass fractions of 0, 0.5%, 1.0%, and 1.5% was added as a sintering aid. The sample numbers were marked as M0, M1, M2, and M3 respectively.
The cement slurry mixer was used for mixing. First, SiC coarse particles and ρ-Al2O3 powder were added and dry mixed for 2min, then SiC powder and BMC were added and dry mixed for 3min, and finally 6% (w) PVA solution was added and mixed for another 3min to make a semi-dry material. The sample was pressed in a steel mold at 50MPa to make a 120mm×20mm×10mm sample. The sample after demoulding was naturally dried for 24h, and then placed in an oven at 110℃ for 24h. Finally, the temperature was raised to 1300-1420℃ in a resistance furnace at 5℃·min-1 and kept warm for 3h.
1.2 Performance test
The TG-DTA curve of basic magnesium carbonate was tested by a German STA449F3 synchronous thermal analyzer with a heating rate of 15℃·min-1; the flexural strength of the sample was tested by a Shanghai CTM6005 press, the fracture morphology of the sample was observed by a Hitachi Hitachi S4800 scanning electron microscope, and the phase composition of the sample was analyzed by a Dutch X-ray diffractometer. Thermal shock test: When the furnace temperature rises to 1050℃, the sample is placed in the furnace, and when the furnace temperature returns to 1050℃, it is kept warm for 20min; then the sample is taken out and quickly placed under a fan for forced cooling to room temperature. This is one thermal shock cycle. The above process was continuously operated for 5 times, and the room temperature flexural strength of the sample was tested. The thermal shock resistance of the sample was evaluated by the room temperature flexural strength retention rate.
2 Results and discussion
2.1 Thermogravimetric analysis of basic magnesium carbonate
Figure 1 shows the TG-DTA curve of BMC. It can be seen that the mass loss mainly occurs at 200-600℃, and the total mass loss rate is about 51.5%; the DTA curve shows three main endothermic peaks, located at 292.9, 382.1 and 571.1℃, corresponding to the removal of crystal water and the removal of hydroxyl and carbon dioxide. BMC is completely decomposed into MgO at 600℃ and participates in the subsequent in-situ reaction.
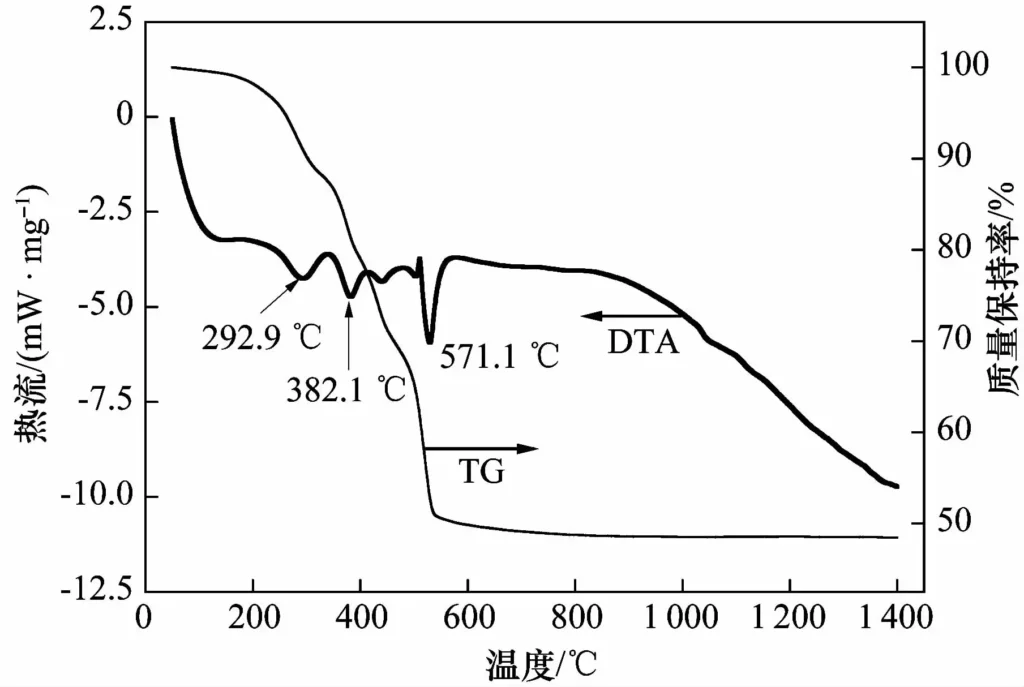
2.2 Phase composition and microstructure of sintered samples
Figure 2 shows the XRD spectrum of samples with different contents of BMC added after sintering at different temperatures. As shown in Figure 2 (a), after sintering at 1300℃, the sample without BMC is mainly composed of SiC, corundum and quartz phases, and no mullite is detected. The quartz phase in the sample is formed by the oxidation product of SiC above 1200℃, and the corundum phase comes from the added ρ-Al2O3. The sample with BMC has a relatively weak mullite peak, and its diffraction intensity increases with the increase of BMC addition. This shows that adding BMC can reduce the mullitization temperature of corundum and quartz phases and promote in-situ reaction sintering.
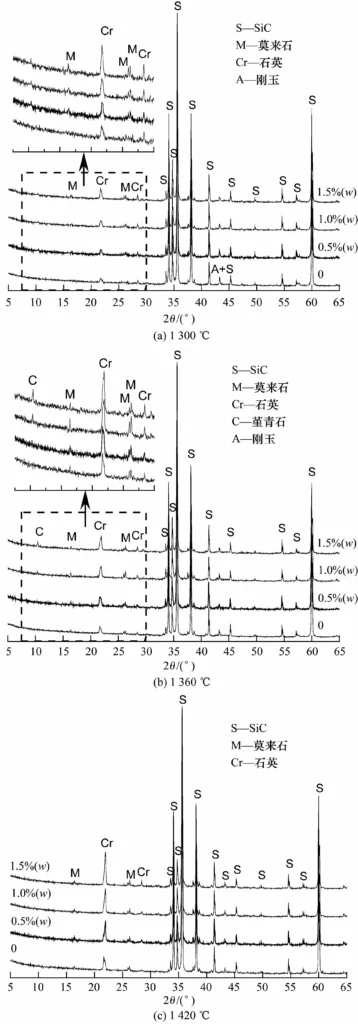
After sintering at 1360℃, the sample with BMC has a cordierite peak, and its diffraction intensity increases with the increase of BMC addition, indicating that the introduced MgO mainly exists in the form of cordierite at this temperature. As can be seen from the enlarged image of Figure 2 (b), a weak mullite peak appeared in the sample without BMC addition, while the mullite peak of the sample with BMC addition was stronger than that at 1300℃, and it increased with the increase of BMC addition. However, when the BMC addition reached 1.5% (w), the diffraction intensity of the mullite peak decreased again. This is because as the BMC content increases, although it is beneficial to mullitization, once the reaction conditions are conducive to the formation of cordierite, more Al2O3 will be consumed, resulting in a decrease in the mullite content. Therefore, adding 1% (w) BMC is beneficial to increasing the mullite content in the binding phase.
As can be seen from Figure 2 (c), after calcination at 1420℃, the mullite peak intensity of each sample is equivalent, indicating that the amount of BMC addition has little effect on it. It is worth mentioning that the cordierite diffraction peak that appeared at 1360℃ for the sample with BMC addition disappears. This indicates that cordierite has decomposed at this temperature, and the generated MgO mainly exists in the form of glass phase, which will have an adverse effect on the mechanical properties of the sample.
It can also be seen from Figure 2 that the sample with BMC addition has a higher quartz phase diffraction intensity than the sample without BMC addition, and it increases with the increase of BMC addition, indicating that the addition of BMC intensifies the oxidation of SiC.
Generally speaking, the mullite transformation between Al2O3 and SiO2 needs to be above 1400℃. When a small amount of BMC is added, the MgO generated after decomposition participates in the formation of a small amount of Mg-Al-Si-O liquid phase at 1300℃ and below, which promotes the nucleation and growth of mullite, and at the same time enhances the diffusion capacity of [O], thereby intensifying the oxidation of SiC. The more BMC is added, the more obvious the sintering effect and the phenomenon of promoting SiC oxidation are. At 1360℃, since the eutectic temperature of the MgO-Al2O3-SiO2 ternary system (1355℃) is exceeded, more liquid phase appears in the bonding phase. The existence of liquid phase greatly improves the diffusion capacity of ions and accelerates the in-situ reaction process. After sintering at 1360℃, the mullite formation of the sample with 1.0% (w) BMC has been basically completed, and the diffraction peak reaches the strongest, while the mullite formation of the sample without BMC has just begun. It can be seen that adding BMC can reduce the mullite formation temperature to 1300℃ and enable the sample to be fully sintered at 1360℃.
Figure 3 shows the fracture morphology of the samples with different amounts of BMC added after sintering at different temperatures. As shown in Figure 3, after sintering at 1300℃, the SiC coarse particles inside the sample without BMC have not formed a clear neck connection, and Al2O3 particles are attached to the surface of SiC particles. However, due to the appearance of a small amount of liquid phase between the particles of the sample with BMC, a binding phase is formed, and it increases with the increase of BMC addition. When 1.5% (w) BMC is added, a relatively intact neck is formed between the particles, making the sample have a higher strength. After sintering at 1360℃, only a few SiC particles are formed inside the sample without BMC, and some Al2O3 particles that do not participate in the reaction are still attached to the surface of SiC particles. However, the sample with BMC is more thoroughly sintered by the sintering aid of MgO, so that the SiC particles are firmly connected together by the newly generated binding phase. Due to the high bonding strength, many SiC particles appear at the fracture of the sample. After sintering at 1420℃, the samples with and without BMC addition have completed sintering and formed a relatively complete neck connection, but the number of SiC transgranular fractures at the fracture surface has decreased. This is due to the excessive presence of quartz phase or glass phase in the bonding phase, which leads to a decrease in bonding strength.
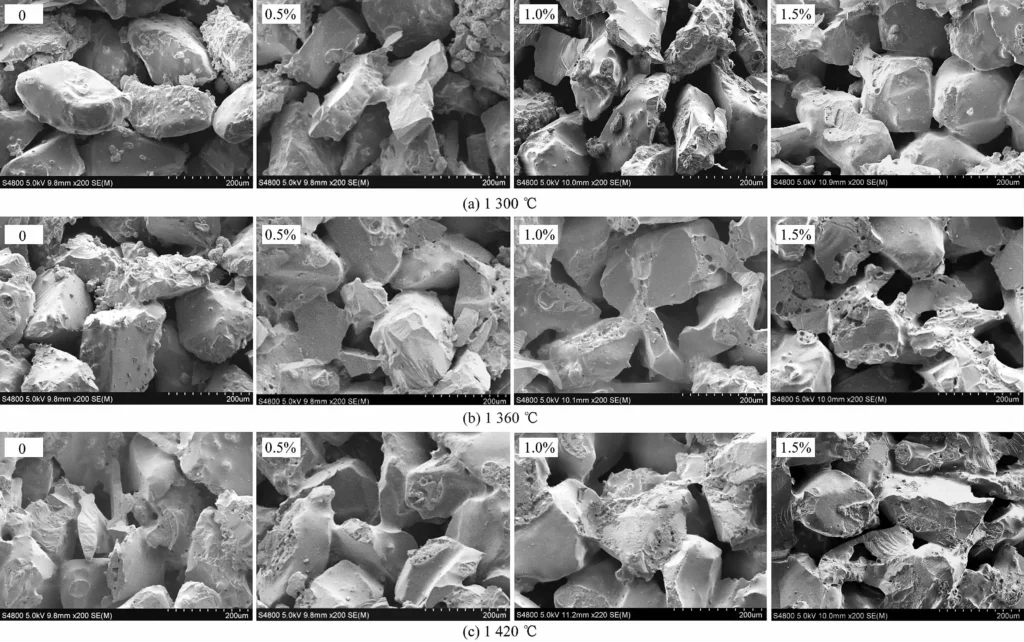
2.3 Performance of sintered samples
Figure 4 shows the strength and apparent porosity of samples with different BMC contents after sintering at 1300, 1360 and 1420℃. The apparent porosity of the sample decreases after sintering, firstly because the densification of the bonding phase makes the gaps between particles smaller (the measured shrinkage value is within 0.2%). Secondly, the volume expansion of 108% is generated after the surface of the SiC particles is oxidized into the quartz phase, which also occupies part of the pore space.
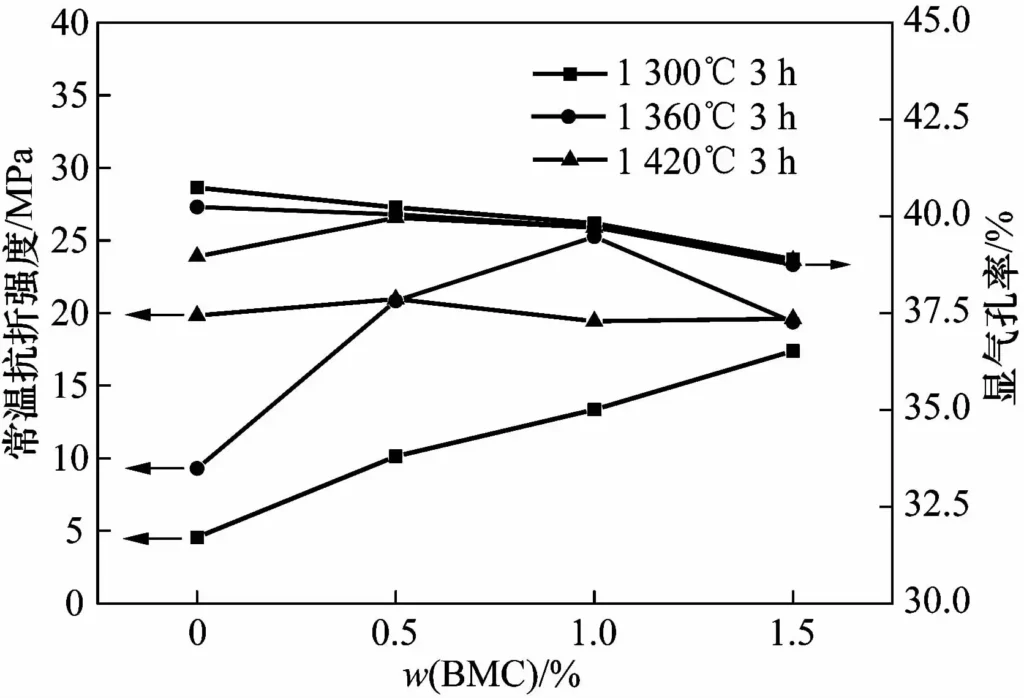
As can be seen from Figure 4, after the samples are sintered at different temperatures, the apparent porosity decreases with the increase of the amount of sintering aid added, while the sintering temperature has little effect on the apparent porosity of the sample. This shows that the content of the sintering aid is the main factor affecting the apparent porosity of the sample.
The room-temperature flexural strength of the sample shows different changes after being sintered at different temperatures. When the BMC addition (w) increases from 0 to 1.5%, the room-temperature flexural strength of the sample after sintering at 1300℃ increases from 4.5MPa to 17.4MPa. After sintering at 1360℃, the room-temperature flexural strength of the sample increases from 9.3MPa to 25.3MPa, and then decreases to 19.4MPa. As mentioned above, this is because the excessive residual quartz phase reduces the strength of the sample. After sintering at 1420℃, the strength of the sample is between 19.0 and 21.0MPa, indicating that the addition of BMC has little effect on it.
In summary, the addition of BMC sintering aid is preferably 1% (w), and the optimal sintering temperature of porous SiC is 1360℃.
Figure 5 shows the room temperature flexural strength and strength retention rate of the sample sintered at 1360℃ (the sample without BMC was sintered at 1420℃) after 5 cycles of thermal shock test at 1050℃. It can be seen that after the thermal shock test, the room temperature flexural strength of the samples with 0.5% and 1.0% (w) BMC addition were 19.8 and 22.8 MPa, respectively, and the corresponding strength retention rates were 97.2% and 90.1%, respectively, indicating that adding a small amount of BMC is beneficial to improving the thermal shock resistance of the support. This is because the presence of MgO increases the mullite content in the binding phase. When the BMC addition amount is 1.5% (w), the strength retention rate is 86.3%, which is lower than that of other samples. This is because the excessive BMC makes the residual quartz content in the binding phase too high, resulting in a decrease in thermal shock resistance. Therefore, the addition of 0.5% and 1.0% (w) BMC can make the reaction-bonded SiC support have better thermal shock resistance.
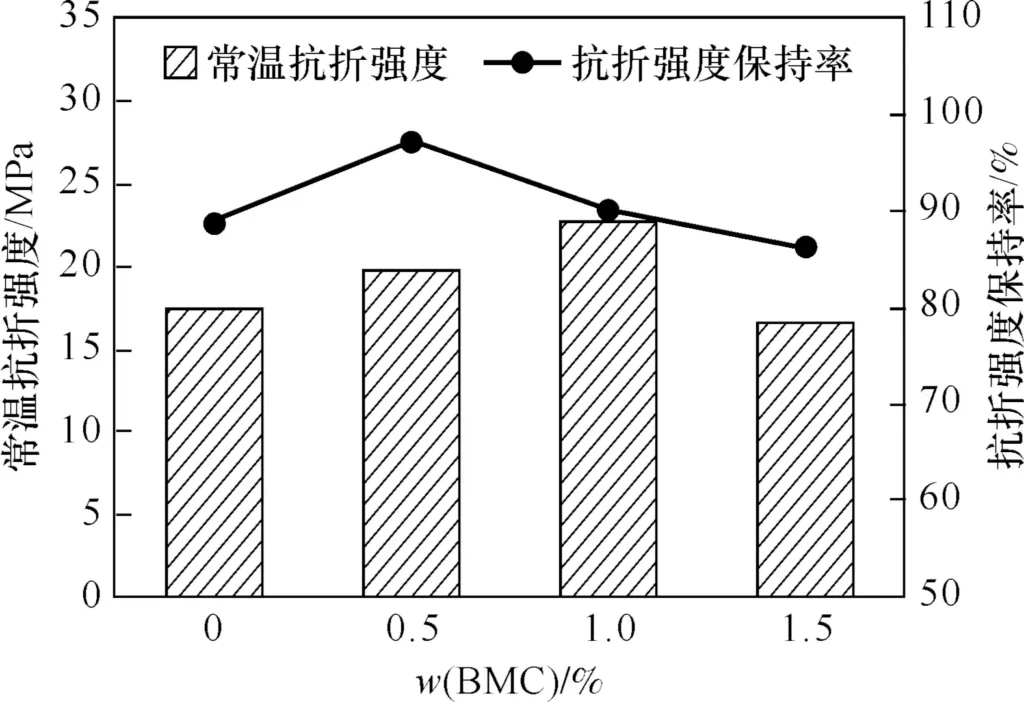
3 Conclusion
(1) Basic magnesium carbonate (BMC) has an obvious sintering effect on the reaction-bonded porous SiC support, which can reduce the sintering temperature to 1300℃, and at the same time promote the oxidation of SiC to generate more quartz phase, which together with the mullite generated by the reaction form a neck connection between SiC particles, making the support have higher strength.
(2) The optimal addition amount of BMC sintering aid is 1.0% (w). The room temperature flexural strength of the porous SiC support prepared after sintering at 1360℃ is 25.3MPa, which is 2.7 times that of the one without sintering aid.
(3) Adding an appropriate amount of BMC sintering aid can improve the thermal shock resistance of the porous SiC support. After adding 0.5% and 1.0% (w) BMC and subjected to 5 wind-cooled thermal shocks at 1050℃, the room-temperature flexural strength retention rate was over 90%, and the room-temperature flexural strength after thermal shock was over 19 MPa, which can meet the index requirements of membrane support for high-temperature flue gas purification.